This Year’s Physics Nobel Awards Scientists for Slicing Reality into Attoseconds
Nobel #Nobel
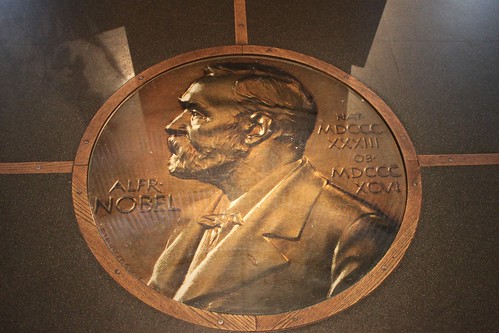
Some processes in physics happen in the blink of an eye while others happen in the blink of a photon. This year’s Nobel Prize in Physics was awarded to Pierre Agostini of the Ohio State University, Ferenc Krausz of the Max Planck Institute of Quantum Optics in Garching, Germany, and Anne L’Huillier of Lund University in Sweden for developing the field of ultraast laser pulses. L’Huillier is only the fifth woman to have ever won the Nobel Prize in Physics.
These pulses are on the scale of the attosecond—a billionth of a billionth of a second. This duration is so short that there are as many attoseconds in a single second as there have been seconds in the entire history of the universe. This year’s prize was awarded “for experimental methods that generate attosecond pulses of light for the study of electron dynamics in matter,” according to a press release from the Royal Swedish Academy of Sciences.
“Attosecond science allows us to address fundamental questions,” said Eva Olsson, chair of the Nobel Committee for Physics at a press conference today. At the atomic level, the motions of electrons and nuclei typically take place over the course of attoseconds. In the late 19th century early photographers made use of cameras to determine whether a horse took all of its hooves off the ground at a gallop—a process too fast for the human eye to discern. (Spoiler: horses do completely leave the ground.) Today’s researchers hope to do the equivalent at attosecond timescales by using ultrafast lasers to get clearer views of otherwise blurry atomic processes.
“When you look at the impact of ultrafast processes, they are inherent to many important mechanisms in life,” says Ursula Keller, a physicist at the Swiss Federal Institute of Technology Zurich. Processes that involve the conversion of photons into electrons, such as photosynthesis and even basic vision, all happen in attosecond time frames. “The dream is really to see electrons move. And I think this is getting closer and closer to reality,” says Carla Faria, a theoretical physicist at University College London.
Attosecond scientists were uniformly excited by the award. “I’m really, really excited and really proud of the people who got the Nobel Prize,” says László Veisz, a physicist at Umeå University in Sweden. Keller agrees and points to L’Huillier in particular. “This is really a woman who absolutely deserves it,” she says. “I hope there will be no more discussion about ‘somebody got a Nobel Prize just because they’re a woman’ or something stupid like this.”
Generating light in extremely short pulses is not easy. For many years light pulses were stuck in the femtosecond regime (one femtosecond is 1000 attoseconds). That’s good enough to resolve molecules in chemical reactions—a feat that won the 1999 Nobel Prize in Chemistry—but it’s insufficient to spot the zigging and zagging of speedier electrons. The problem was fundamental: even the briefest physically achievable optical laser pulse was a few femtoseconds in length. “You cannot generate a pulse [that] is shorter than one wavelength,” says Mauro Nisoli, a physicist at the Polytechnic University of Milan in Italy. So to get past the femtosecond barrier, physicists needed to produce light with shorter wavelengths.
One way to do that is a process called high-harmonic generation (HHG), in which an electron absorbs several low-energy photons and spits out a single high-energy photon. But decades ago HHG seemed to offer diminishing returns, with the number of photons that were emitted decreasing as the energy went up and eventually dwindling away. Then, in 1987, L’Huillier and her colleagues fired an infrared laser through argon and saw something fascinating: instead of decreasing as energy increased, the number of emitted photons remained steady. “What Anne L’Huillier discovered is this plateau,” Keller says. “And it was really a game changer.”
Within a few years L’Huillier and others in the field worked out what was happening in such specialized HHG setups. The electrons in argon were performing a complex, three-step dance, first tunneling quantum mechanically away from the atom, then accelerating away from it and finally falling back into its embrace to release their energy as a high-energy photon. This would happen multiple times during an initiating laser pulse and lead to a train of ultrafast, attosecond-scale flashes of light from the gas.
Going from L’Huillier’s work on HHG to a working attosecond source required two key innovations. First, researchers had to measure the pulse timings, and second, they had to generate an single isolated pulse. Typically, when lasers need to be timed, they are measured with a shorter laser pulse. “How do you measure the duration of something that’s the shortest time length?” Veisz asks rhetorically. The answer is that you must measure it with itself, he says. One technique that uses this principle is called frequency-resolved optical gating (FROG), which is unusable for attosecond pulses because they’re too low-energy.
Building off of FROG, Agostini created an approach called RABBIT (reconstruction of attosecond beating by interference of two-photon transitions), which works by combining the electric field of the optical laser with the attosecond pulses. (Laser techniques are often named after animals, Veisz says.) Meanwhile Krausz independently developed a similar method for his single pulses called attosecond streaking. Able to characterize the timing of the shortest pulses in the world, researchers now had attosecond sources with which to see the universe on a previously unimaginable timescale.
With the newfound probes developed by Agostini, Krausz and L’Huillier, researchers can now generate laser pulses of merely a few dozen attoseconds. Further refinements of these approaches to generate ever shorter pulses promise to deepen scientists’ understanding of electron dynamics and applications. Nisoli points out that while femtosecond lasers can be used to closely monitor chemical reactions, attosecond pulses are so precise that they can be used to nudge the electrons themselves, potentially eliciting a shift from passive observation to active control of chemistry on unprecedented scales. Attosecond pulses can even control the properties of solids, turning an insulator into a conductor and back again in a flash.
There are more fundamental possibilities, too, such as more detailed explorations of Einstein’s famed photoelectric effect, in which a photon impinges on metal, causing the metal to emit an electron. “Everybody thought that this is instantaneous, and attosecond physics showed it is not, and this triggered a lot of theoretical studies,” Veisz says.
As usual, the award came as a surprise to its recipients. When L’Huillier was notified, she was in the middle of giving a lecture and missed the first few calls from Stockholm. After stepping outside to take the call, she returned to the lecture, where she continued teaching without telling her students anything. “Teaching is very, very important. For me, it’s very important,” she told Hans Ellegren, secretary-general of the Royal Swedish Academy of Sciences, over the phone during the prize’s announcement.
Editor’s Note (10/3/23): This story has been updated.